
Droplets or puddles tend to freeze from the propagation of a single freeze front. In contrast, videographers have shown that as soap bubbles freeze, a plethora of growing ice crystals can swirl around in a beautiful effect visually reminiscent of a snow globe. However, the underlying physics of how bubbles freeze has not been studied. Here, we characterize the physics of soap bubbles freezing on an icy substrate and reveal two distinct modes of freezing. The first mode, occurring for isothermally supercooled bubbles, generates a strong Marangoni flow that entrains ice crystals to produce the aforementioned snow globe effect. The second mode occurs when using a cold stage in a warm ambient, resulting in a bottom-up freeze front that eventually halts due to poor conduction along the bubble. Blending experiments, scaling analysis, and numerical methods, the dynamics of the freeze fronts and Marangoni flows are characterized. Click here to read more!
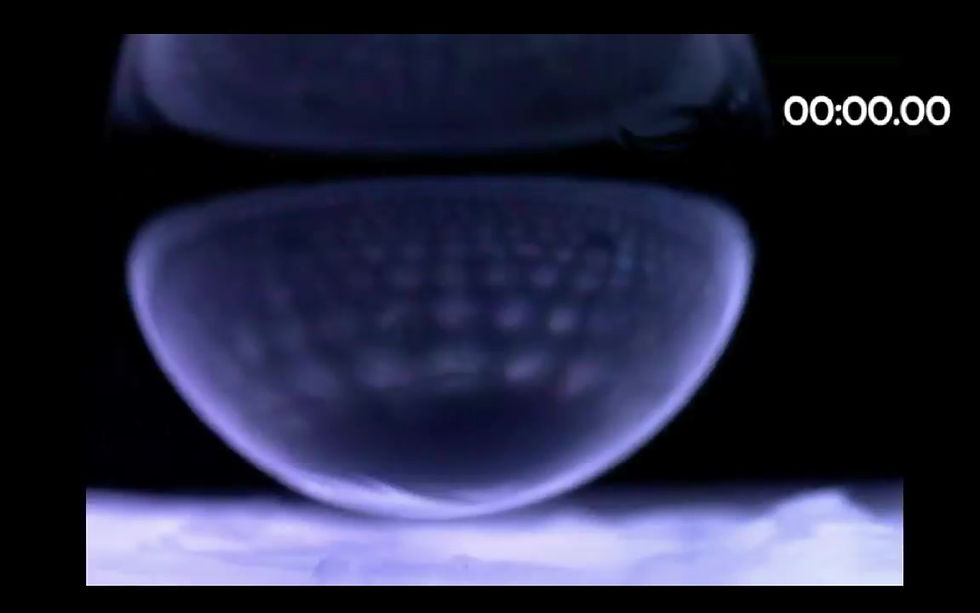
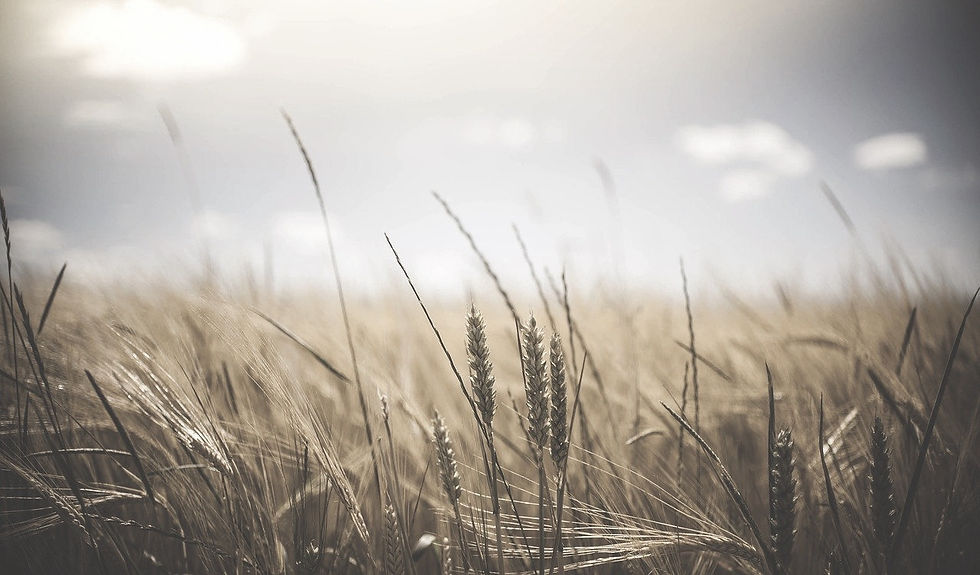

The spread of disease among plants is attributed to strong winds and rainsplash, which are known to liberate and disperse pathogenic spores. We show that naturally occurring dew cycles are a third mechanism for liberating pathogenic material, particularly for superhydrophobic organisms. Using wheat leaves as a case study, high-speed imaging revealed that microscopic dew droplets are able to spontaneously jump several millimeters into the air upon coalescing with neighboring dew. For wheat leaves infected with rust, jumping droplets carried adhered spores with them to heights exceeding that of a typical boundary layer, to enable subsequent dispersal in the wind. These findings indicate that surface wettability and dew cycles play an important role in the spread of disease among wheat crops. The self-cleaning properties of superhy- drophobic wheat leaves are therefore simultaneously responsible for pathogen transport to neighboring plants, analogous to sneezing. Click here to read more!
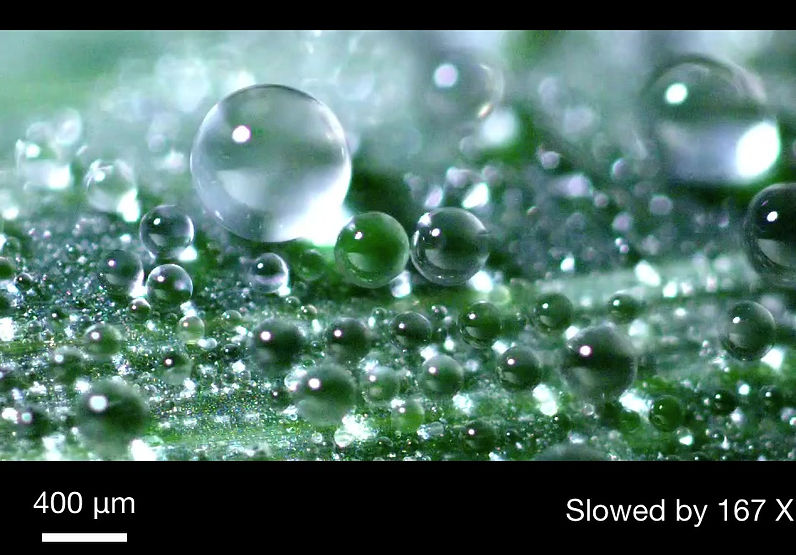
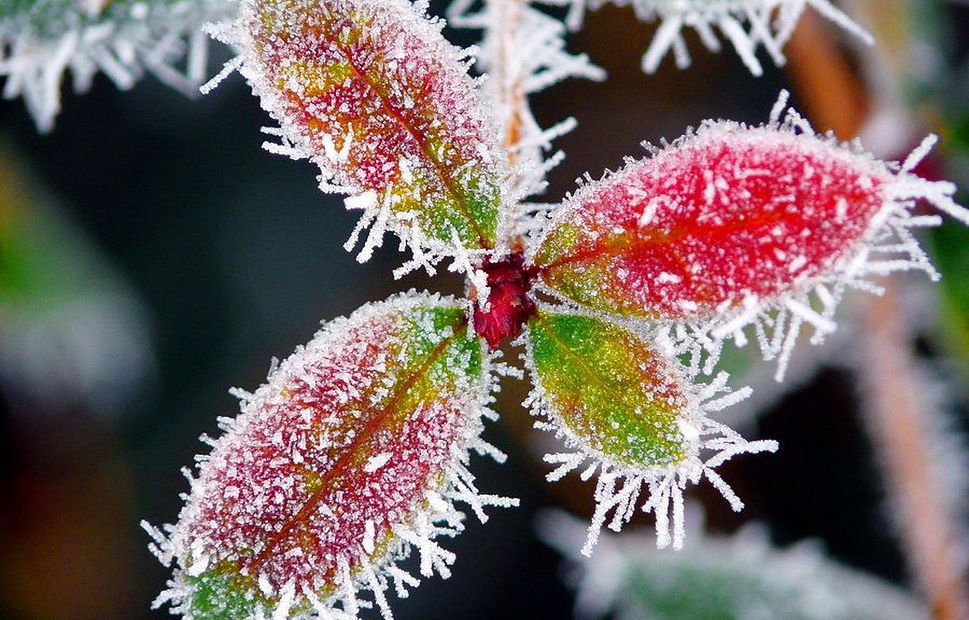
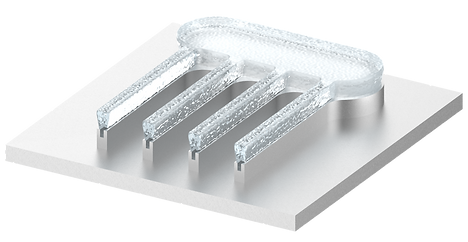
When the temperature of a surface is subfreezing and beneath the dew point, frost grows uncontrollably across the entire surface. Here, we show that in-plane frost growth can be passively suppressed by patterning arrays of microscopic ice stripes across a surface. The vapor pressure of ice is depressed, such that the ice stripes siphon nearby moisture from the atmosphere to keep the intermediate surface regions completely dry of dew or frost. The stripes of frozen water were enabled by fabricating wicking grooves that do not require any surface functionalization, making this approach durable, scalable, and adaptable to virtually any material choice. To adapt the common expression to “fight fire with fire,” perhaps it is time to “fight ice with ice.”. Click here to read more!
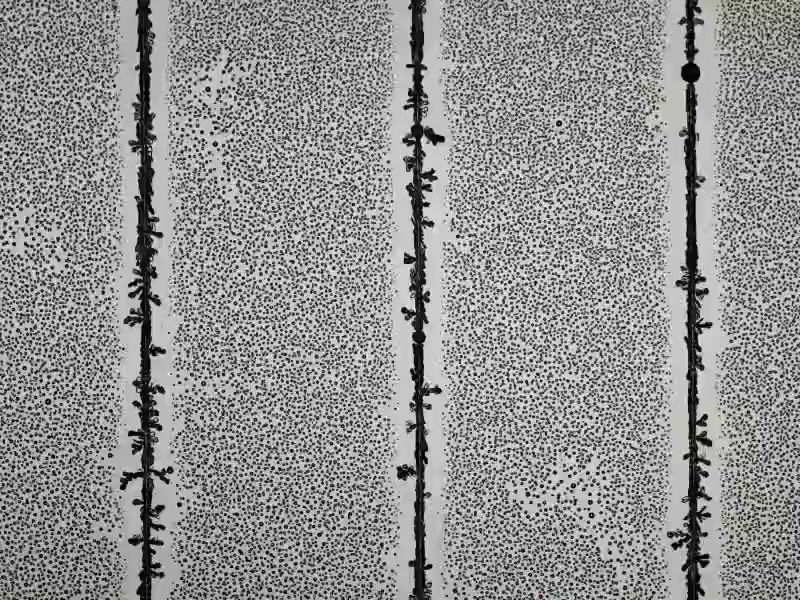
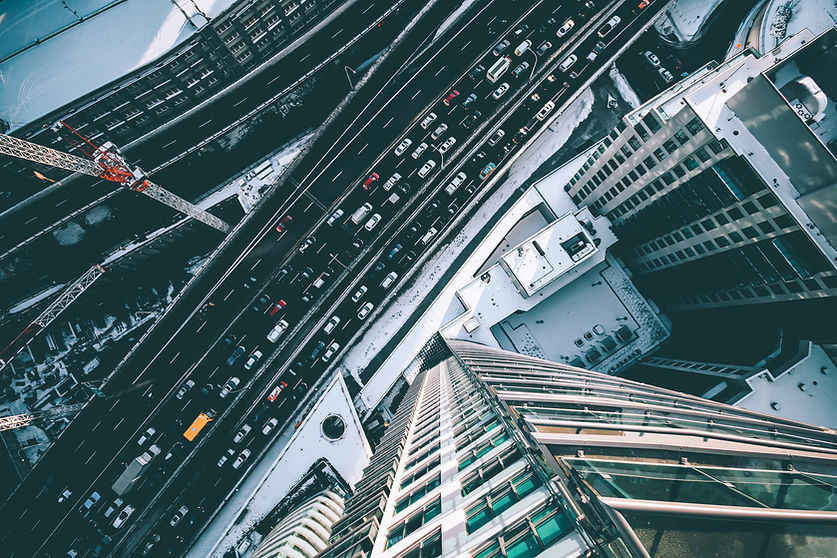
How close should the cars get to each other so that when the light turns green, they can all clear the intersection as efficiently as possible? In this study, we found the answer to this question by recruiting 10 volunteer drivers and systematically varying the packing density of their cars queued at a red light. The study was performed at the Virginia Tech Transportation Institute, where we had full control over the traffic light and the driving conditions. For each packing density, when the light turned green a drone flying overhead captured footage of the resulting flow dynamics. Experimentally, a drone camera captured the dynamics of a queue of cars accelerating from rest through a traffic intersection on a Smart Road. The flow efficiency through the green light remained constant even as the initial bumper-to-bumper spacing between the cars at rest was increased by over a factor of 20. While cars queued in close-packed “solid phase” configurations obviously need to drive a shorter distance to cross the intersection, this advantage was completely offset by the lag time inherent to changing back into a loose-packed “liquid phase” for flow to safely resume (latent heat). This surprising finding challenges the long- standing convention of drivers inducing phase transitions at traffic stoppages, as we show that cars should maintain their regular spacing for increased safety with no loss in efficiency. Finally, motion-capture experiments with pedestrian traffic reveal that latent heat becomes negligible for slower-moving systems, which should indeed utilize close-packed queues. Click here to read more!

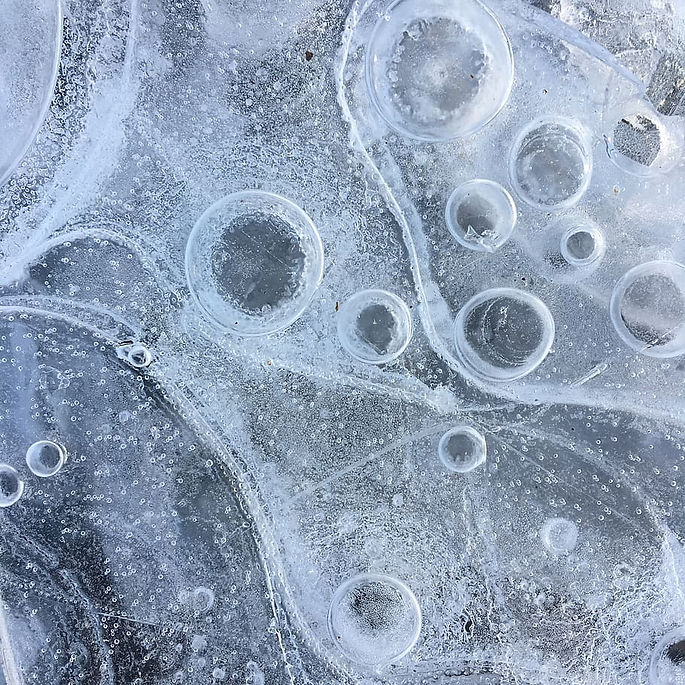


‘Sneezing’ Plants
Liquid-ice interactions are becoming increasingly relevant for many applications including hydraulic fracturing, arctic drilling, and liquid-impregnated surfaces. It is therefore surprising that the capillary action of a liquid wicking across the surface of ice has never been characterized. We observe silicone oil as it wicks up sheets of frost grown on vertically oriented aluminum surfaces of varying wettability. Superhydrophilic and hydrophilic surfaces promote the growth of continuous frost sheets which displace oil with a power-law slope of 1/3 with respect to time. While in contrast to the 1/2 slope of Washburn's law governing most porous media, the 1/3 slope can be rationalized by considering the dendritic frost structure as an array of vertical wedges incapable of supporting the liquid's weight. Nonwetting surfaces grow frost from a more dilute distribution of frozen dropwise condensate, resulting in lower slopes of 1/4 for hydrophobic surfaces and nearly zero for superhydrophobic surfaces promoting jumping-droplet condensation. When switching to a near-horizontal orientation, Washburn's law is obtained for continuous frost sheets. The spreading rate of a liquid across frost can therefore be controlled by tuning the underlying surface wettability or surface orientation. Finally, we show that oils cannot wick inside of a bulk volume of frozen water due to the small pore size of the hexagonal lattice structure. Click here to read more!

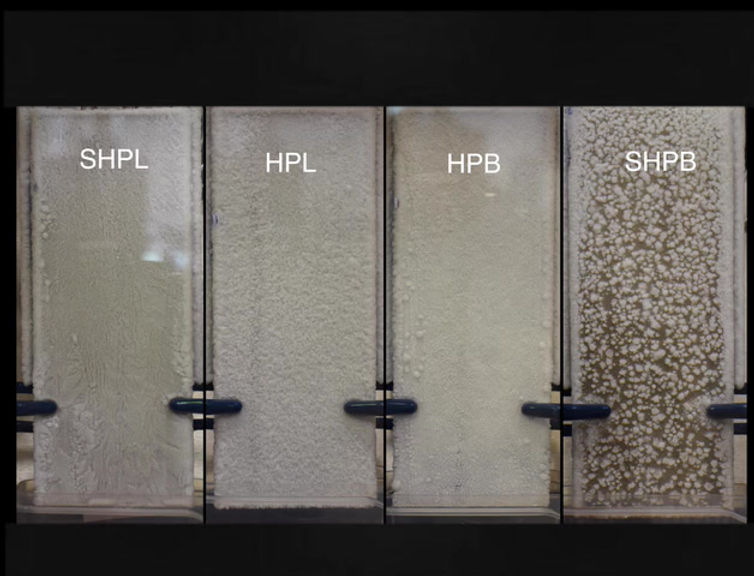